ISL6534 Intersil Corporation, ISL6534 Datasheet - Page 18
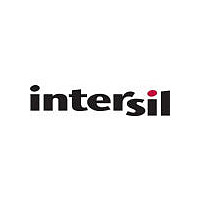
ISL6534
Manufacturer Part Number
ISL6534
Description
Dual PWM
Manufacturer
Intersil Corporation
Datasheet
1.ISL6534.pdf
(26 pages)
Available stocks
Company
Part Number
Manufacturer
Quantity
Price
Company:
Part Number:
ISL6534CR
Manufacturer:
INTERSIL
Quantity:
1 440
Company:
Part Number:
ISL6534CR-T
Manufacturer:
PHILIPS
Quantity:
3 123
Part Number:
ISL6534CR-T
Manufacturer:
INTERSIL
Quantity:
20 000
Company:
Part Number:
ISL6534CRZ
Manufacturer:
TI
Quantity:
7 155
Part Number:
ISL6534CRZ
Manufacturer:
ISL
Quantity:
20 000
Company:
Part Number:
ISL6534CRZ-T
Manufacturer:
INTERSIL
Quantity:
11 900
Part Number:
ISL6534CRZ-T
Manufacturer:
INTERSIL
Quantity:
20 000
Company:
Part Number:
ISL6534CRZR5229
Manufacturer:
Intersil
Quantity:
135
Part Number:
ISL6534CV
Manufacturer:
INTERSIL
Quantity:
20 000
Part Number:
ISL6534CV-T
Manufacturer:
INTERSIL
Quantity:
20 000
www.DataSheet4U.com
COMPENSATION BREAK FREQUENCY EQUATIONS
Figure 16 shows an asymptotic plot of the DC-DC
converter’s gain vs. frequency. The actual Modulator Gain
has a high gain peak do to the high Q factor of the output
filter and is not shown in Figure 16. Using the above
guidelines should give a Compensation Gain similar to the
curve plotted. The open loop error amplifier gain bounds the
compensation gain. Check the compensation gain at F
with the capabilities of the error amplifier. The Closed Loop
Gain is constructed on the log-log graph of Figure 16 by
adding the Modulator Gain (in dB) to the Compensation Gain
(in dB). This is equivalent to multiplying the modulator
transfer function to the compensation transfer function and
plotting the gain.
The compensation gain uses external impedance networks
Z
loop. A stable control loop has a gain crossing with -
20dB/decade slope and a phase margin greater than 45
Include worst case component variations when determining
phase margin.
F
F
1. Pick Gain (R2/R1) for desired converter bandwidth
2. Place 1
3. Place 2
4. Place 1
5. Place 2
6. Check Gain against Error Amplifier’s Open-Loop Gain
7. Estimate Phase Margin - Repeat if Necessary
FB
Z1
Z2
FIGURE 16. ASYMPTOTIC BODE PLOT OF CONVERTER
100
-20
-40
-60
80
60
40
20
(~75% F
0
and Z
=
=
----------------------------------
2π R
----------------------------------------------------- -
2π
10
(R2/R1)
20LOG
•
•
IN
MODULATOR
(
ST
ND
ST
ND
R1
1
LC
to provide a stable, high bandwidth (BW) overall
2 C1
100
GAIN
•
Zero Below Filter’s Double Pole
Pole at the ESR Zero
)
Zero at Filter’s Double Pole
Pole at Half the Switching Frequency
+
1
R3
GAIN
) C3
•
1K
F
Z1
F
FREQUENCY (Hz)
LC
F
Z2
10K
18
F
F
F
P1
F
P1
P2
(V
ESR
IN
100K
=
=
20LOG
F
/
∆
----------------------------------
2π R3 C3
P2
------------------------------------------------------ -
2π R2
V
OSC
•
•
OPEN LOOP
ERROR AMP GAIN
1
1M
)
•
COMPENSATION
GAIN
•
1
CLOSED LOOP
GAIN
--------------------- -
C1
C1 C2
10M
•
+
C2
P2
o
.
ISL6534
FET Selection (VOUT1, VOUT2)
The typical FET expected to be used will have a low r
(5-10mΩ) and a low Vgs (Gate-to-source threshold voltage;
1-2V). It can be packaged in a thermally enhanced SO-8 IC
package (where the drain leads are thermally connected to
the leadframe under the die, or similar approaches), or even
in more conventional power packages (D-PAK). If the FETs
are surface mounted to the PCB, with only the area of the
power planes to conduct the heat away, then the maximum
load current will be limited by the thermal ratings under those
conditions. Using conventional heatsinks or sufficient airflow
can extend the limit of dissipation.
FETs can be paralleled for higher currents; this spreads the
heat between the FETs, which helps keep the temperature
lower. However, the gate driver is now driving twice the gate
capacitance, so there will be more dissipation in the ISL6534
gate drivers.
Typical values for maximum current (based on 8-pin SOIC
FETs surface-mounted on PCB, with no heatsinks or airflow)
are 5A for a dual FET; 10A for single FETs for upper and
lower; and 20A for two FETs in parallel for both upper and
lower. These are just rough numbers; many factors affect it,
such as PCB board area available for heatsinking planes,
how close other dissipative devices are, etc.
In general (and especially for short UGATE duty cycles, such
as converting 12V input down to 1V or 2V outputs), the
upper FET should be chosen to minimize the Gate charge,
since switching losses dominate. Since the lower FET is on
most of the time, low r
consideration.
The ISL6534 requires 2 N-Channel power MOSFETs for
each switcher output. These should be selected based upon
r
management requirements. The following are some
additional guidelines.
In high-current applications, the MOSFET power dissipation,
package selection and heatsink are the dominant design
factors. The power dissipation includes two loss
components; conduction loss and switching loss. The
conduction losses are the largest component of power
dissipation for both the upper and the lower MOSFETs.
These losses are distributed between the two MOSFETs
according to duty factor (see the equations below). Only the
upper MOSFET has switching losses, since the FET body
diode (or optional external Schottky rectifier) clamps the
switching node before the synchronous rectifier turns on.
P
Where: D is the duty cycle = V
P
DS(ON)
LOWER
UPPER
t
Fs is the switching frequency.
, gate supply requirements, and thermal
SW
= I
= I
O
O
is the switching interval, and
2
2
x r
x r
DS(ON)
DS(ON)
DS(ON)
x D + 1
x (1 - D)
should be the main
O
2
/V
IN
Io x V
,
IN
x t
SW
x Fs
DS(ON)
FN9134.1